Among my most salient memories from university is the feeling of panic that consumed me three weeks into my third year. I was taking five heavy courses and three labs, which felt like playing a high stakes game of “Whac-A-Mole” for weeks on end. I was academically drowning, and couldn’t keep my head above water. But If I wanted to get into grad school, I needed the grades. I had no choice but to figure it out. Upon reaching peak levels of despair, I devised a plan. I would cut my sleep in half, waking up each morning at 4:30 a.m. to study for five hours or so before class. I would get much of my work done before I would have normally gotten out of bed. A couple of weeks of my new routine were successful. I found the early mornings incredibly peaceful. I felt less distracted, and started catching up. Midterms came and went, and I appeared to be salvaging my semester. In the midst of this routine, though, I noticed something unusual: my nutrition habits took a sharp turn for the worse. As someone who is pathologically obsessed with nutrition, this pissed me off. I knew what to eat, but I couldn’t force myself to eat it. Black coffees were replaced by sugary lattes. Salads were replaced by poutine at the campus pub. I felt physically softer, possessed by an inner demon intent on stealing my discipline and giving me metabolic disease. Ever since that semester, I’ve been intensely curious about the link between sleep and nutrition. Did sleep deprivation play a role in the erosion of my discipline? If so, how? What changed psychologically, hormonally, or neurochemically?
A semester later, I took a course on molecular endocrinology which helped me answer my question. We began our unit on nutrition by examining two fascinating hormones: ghrelin and leptin. In 2000, a paper in Nature 1 showed that injecting ghrelin into the cerebral ventricles (fluid-filled cavities which bathe the brain) of rodents elicited a linear increase in their food intake. It also found serum ghrelin levels to be higher in fasted rats than well fed rats. Researchers classified ghrelin as an orexigenic hormone. It makes you hungry. How does it do this? By taking electrical recordings from a subset of neurons in the limbic system, scientists demonstrated that ghrelin acts on the hypothalamus to presynaptically enhance the neuropeptide release of two further orexigenic hormones called neuropeptide Y (NPY) and Agouti-related peptide (AgRP) 2 3. NPY and AgRP send outgoing signals to activate the parasympathetic (rest and digest) nervous system, increasing food intake and stimulating anabolism (growth). Ghrelin also inhibits the release of a neuropeptide called Cocaine and Amphetamine-Regulated Transcript (CART)4. CART usually acts to decrease food intake, so ghrelin’s inhibition of CART stimulates appetite. The other hormone I initially mentioned, leptin, is the yin to ghrelin’s yang. Leptin is an anorexigenic hormone. It is constitutively released by fat cells to make you feel full5. It modulates the same downstream neurons as ghrelin, affecting them in precisely the opposite way. Leptin inhibits NPY and AgRP, and stimulates CART, thereby synergistically decreasing food intake6.
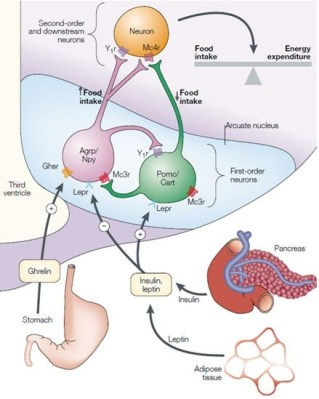
Figure 1. Some of the hormonal interactions described above are shown.
Though this is a fairly pathetic, low resolution depiction of the endocrinology behind appetite regulation, it will suffice for now. When your body needs calories, it produces more ghrelin and less leptin, which makes you feel hungry. When your body needs no further calories, it produces more leptin and less ghrelin, which makes you feel full. Once I had a basic grasp of the hormonal basis for appetite control, I began to wonder how these hormones might relate to my sleep deprivation experience.
My research led me to an observational epidemiology study published in 2004 which reported associations between sleep duration, ghrelin levels, leptin levels, and body mass index. Evidently, those who slept the least had the highest concentrations of ghrelin, the lowest concentrations of leptin, and the highest body mass indices7. This pattern was convincing, and made sense mechanistically. Ghrelin makes you hungry. Leptin makes you full. Sleep deprivation causes ghrelin levels to rise, and leptin levels to plummet. Though I thought I had my answer, my statistical conscience reminded me of the inherent problem with epidemiology: it cannot be used to distinguish cause from effect. This calls for a brief digression: the scientific method proceeds by way of observation, hypothesis, and experimentation. Due to its inherent lack of control, there is one component of this sequence that epidemiology will always lack: experimentation. Without controlling for potentially confounding variables, it is impossible to distinguish between cause and coincidence. Thankfully, a researcher at the University of Chicago named Eve Van Couter came to the rescue, performing a randomized, controlled experiment examining the effects on sleep deprivation on leptin levels 8. She brought participants into her sleep lab and restricted them to six hours of sleep per night for six days, followed by nine hours of sleep per night for the next six days. Critically, she ensured that caloric intake was the same for all participants. Leptin levels were measured continuously for the 12 days. As it turns out, leptin concentrations were 20% lower in the sleep deprived state than in the sleep extended state. This begins to explain my predicament during third year. Leptin tells you you’re full. Sleep deprivation decreased my leptin. Ergo, when I cut my sleep in half, my inner fat kid was unleashed. And he was eating with a vengeance.
That was leptin. What about ghrelin? The same lab solved that puzzle as well, demonstrating that a similar sleep deprivation protocol caused a 20% increase in ghrelin concentration. 9. Increased hunger accompanied this hormonal shift. I had my answer, I figured. Sleep deprivation elicits hormonal chaos. When I was sleep deprived, leptin tanked, ghrelin skyrocketed, and I started making bad decisions.
Though I thought I had a clear idea of what caused my predicament, I subsequently came across a study which highlighted the frightening insufficiency of my explanation. In 2012, Eve Van Cauter’s lab ran a study examining the effects of sleep deprivation on insulin sensitivity. Before the experiment, healthy volunteers were administered eu-glycemic insulin clamps (the gold standard for measuring glucose disposal, the rate at which peripheral tissues take up glucose from the blood). Following this initial measurement, their sleep was restricted to four hours per night for 14 days. After those two weeks, the volunteers were administered a second eu-glycemic clamp. The post-intervention numbers are insane: glucose disposal efficiency had decreased by fifty percent. 10. Put differently, sleep deprived participants became fifty percent less sensitive to insulin. To appreciate the gravity of this discovery, some background on glucose homeostasis is needed.
There are certain physiological imperatives. One is this: blood glucose levels must stay within a narrow range. If they rise above a certain threshold, the consequences are dire (e.g. kidney disease11, retinopathy12, ketoacidosis13). If they drop below a certain threshold, the consequences are similarly terrible (e.g. seizures14, loss of consciousness15, death16). When we consume carbohydrates, our body degrades them into glucose. If glucose levels rise above threshold, beta cells in the pancreas secrete insulin into the bloodstream. Insulin then binds to receptors on muscle, liver, and fat cells which recognize its unique biochemical signature. Insulin’s binding triggers a cascade of biochemical events (mostly phosphorylations) which culminate in the translocation of a glucose transporter into the cell membrane. The channel (called GLUT 4) creates thermodynamically favourable conditions for the passage of the polar (think water) glucose through the non-polar (think oil) membrane. Once inside a muscle or liver cell, glucose is either converted to glycogen (a storage molecule from which cellular energy can later be derived) or oxidized to generate ATP (the cell’s energy currency)17.
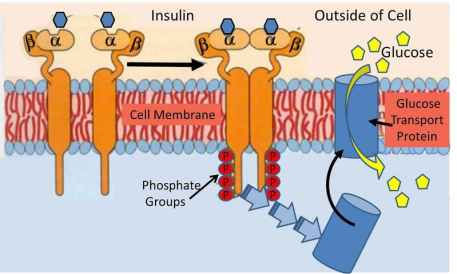
Figure 2. Insulin is shown binding to its receptor, and triggering a cascade of phosphorylations which elicit the translocation of a glucose transporter into the cell membrane.
But here’s the thing. Glucose is converted to glycogen in limited amounts. If cells become resistant to insulin (as they did in the sleep deprived volunteers), they lose the ability to use glucose to trigger glycogen synthesis or to generate energy. In this scenario, insulin elicits the conversion of glucose to triglycerides (fat) in unlimited amounts. In fact, adipose tissue appears uniquely immune from insulin resistance 18. Sadly, fat storage can persist forever. This probably made sense for most of our evolutionary history, when food was scarce. In our modern environment of relative abundance, however, perpetual fat storage is maladaptive. This is one of many mismatches between our evolved biology and our current environment. To further exacerbate the situation, insulin tells adipocytes to inhibit an enzyme called hormone sensitive lipase19. This enzyme usually degrades fat. But when muscle and liver cells lose sensitivity to insulin, and insulin is free to bind to receptors in adipose tissue, fat ceases to be burned, and is even more efficiently stored. This is the perfect metabolic storm. Not only does sleep deprivation wreak havoc on appetite regulation, but it reduces the efficiency with which you can mitigate the metabolic damage it causes you to incur.
This story illustrates something I’ve been thinking about a lot lately. Effective solutions to intractable problems are often counterintuitive. How should people improve their nutrition habits? The obvious answer is that they should “just eat better.” But if it was that easy, wouldn’t everyone just do it? We all know how to eat well, more or less. The rate limiting step is compliance. So what’s the solution? Exert more discipline? Just suck it up and stop giving into your temptations? Well, maybe. A daily routine borne of hard fought discipline is what ultimately sustains you. But why swim upstream? Why not make it easier on yourself? Why not attack the problem obliquely, from multiple angles? Optimize your sleep, and you might just unknowingly optimize your nutrition.
I plan to write more about sleep, as its effects on health seem endless. I’ll likely explore other interesting side effects of sleep deprivation, and finish off this series with a research review on optimal sleep hygeine practices. In the meantime…sleep well, my friends.
Tschöp, M., Smiley, D. L., & Heiman, M. L. (2000). Ghrelin induces adiposity in rodents. Nature, 407(6806), 908.
Cowley, M. A., Smith, R. G., Diano, S., Tschöp, M., Pronchuk, N., Grove, K. L., … & Garcia-Segura, L. M. (2003). The distribution and mechanism of action of ghrelin in the CNS demonstrates a novel hypothalamic circuit regulating energy homeostasis. Neuron, 37(4), 649-661.
Kamegai, J., Tamura, H., Shimizu, T., Ishii, S., Sugihara, H., & Wakabayashi, I. (2001). Chronic central infusion of ghrelin increases hypothalamic neuropeptide Y and Agouti-related protein mRNA levels and body weight in rats. Diabetes, 50(11), 2438-2443.
de Lartigue, G., Dimaline, R., Varro, A., & Dockray, G. J. (2007). Cocaine-and amphetamine-regulated transcript: stimulation of expression in rat vagal afferent neurons by cholecystokinin and suppression by ghrelin. Journal of Neuroscience, 27(11), 2876-2882.
Keim, N. L., Stern, J. S., & Havel, P. J. (1998). Relation between circulating leptin concentrations and appetite during a prolonged, moderate energy deficit in women. The American journal of clinical nutrition, 68(4), 794-801.
Kristensen, P., Judge, M. E., Thim, L., Ribel, U., Christjansen, K. N., Wulff, B. S., … & Larsen, P. J. (1998). Hypothalamic CART is a new anorectic peptide regulated by leptin. Nature, 393(6680), 72.
Taheri, S., Lin, L., Austin, D., Young, T., & Mignot, E. (2004). Short sleep duration is associated with reduced leptin, elevated ghrelin, and increased body mass index. PLoS medicine, 1(3), e62.
Spiegel, K., Leproult, R., L’Hermite-Balériaux, M., Copinschi, G., Penev, P. D., & Van Cauter, E. (2004). Leptin levels are dependent on sleep duration: relationships with sympathovagal balance, carbohydrate regulation, cortisol, and thyrotropin. The Journal of clinical endocrinology & metabolism, 89(11), 5762-5771.
Spiegel, K., Tasali, E., Penev, P., & Van Cauter, E. (2004). Brief communication: sleep curtailment in healthy young men is associated with decreased leptin levels, elevated ghrelin levels, and increased hunger and appetite. Annals of internal medicine, 141(11), 846-850.
Broussard, J. L., Ehrmann, D. A., Van Cauter, E., Tasali, E., & Brady, M. J. (2012). Impaired insulin signaling in human adipocytes after experimental sleep restriction: a randomized, crossover study. Annals of internal medicine, 157(8), 549-557.
Amri, K., Freund, N., Vilar, J., Merlet-Benichou, C., & Lelievre-Pegorier, M. (1999). Adverse effects of hyperglycemia on kidney development in rats: in vivo and in vitro studies. Diabetes, 48(11), 2240-2245.
Klein, R., Klein, B. E., Moss, S. E., & Cruickshanks, K. J. (1994). Relationship of hyperglycemia to the long-term incidence and progression of diabetic retinopathy. Archives of internal medicine, 154(19), 2169-2178.
Kerl, M. E. (2001). Diabetic ketoacidosis: pathophysiology and clinical and laboratory presentation. Compendium, 23(3), 220-228.
Malouf, R., & Brust, J. C. (1985). Hypoglycemia: causes, neurological manifestations, and outcome. Annals of Neurology: Official Journal of the American Neurological Association and the Child Neurology Society, 17(5), 421-430.
Cryer, P. E. (1999). Symptoms of hypoglycemia, thresholds for their occurrence, and hypoglycemia unawareness. Endocrinology and metabolism clinics of North America, 28(3), 495-500.
Cryer, P. E. (1999). Symptoms of hypoglycemia, thresholds for their occurrence, and hypoglycemia unawareness. Endocrinology and metabolism clinics of North America, 28(3), 495-500.
Sesti, G. (2006). Pathophysiology of insulin resistance. Best practice & research Clinical endocrinology & metabolism, 20(4), 665-679.
Mizuno, T. M., Funabashi, T., Kleopoulos, S. P., & Mobbs, C. V. (2004). Specific preservation of biosynthetic responses to insulin in adipose tissue may contribute to hyperleptinemia in insulin-resistant obese mice. The Journal of nutrition, 134(5), 1045-1050.
STRÅLFORS, P., & Honnor, R. C. (1989). Insulin‐induced dephosphorylation of hormone‐sensitive lipase: Correlation with lipolysis and cAMP‐dependent protein kinase activity. European journal of biochemistry, 182(2), 379-385.
Comments